System Management Interrupts (SMI) provide a mechanism for entering System Management Mode (SMM) which primarily implements platform-specific functions related to power management. SMM is a privileged execution mode with access to the complete physical memory of the system, and to which the operating system has no visibility. This makes the code running in SMM an ideal target for malware insertion and potential supply chain attacks. Accordingly, it would be interesting to develop a mechanism to audit the SMIs present on a running system with the objective of cross-referencing this information with data provided by the BIOS supplier. This could help ensure that no new firmware entry-points have been added in the system, particularly in situations where there is either no signature verification for the BIOS, or where such verification can be bypassed by the attacker.
The section 32.2, “System Management Interrupt (SMI)” of Intel’s System Programming Guide [1], states the following regarding the mechanisms to enter SMM and its assigned system priority:
“The only way to enter SMM is by signaling an SMI through the SMI# pin on the processor or through an SMI message received through the APIC bus. The SMI is a nonmaskable external interrupt that operates independently from the processor’s interrupt- and exception-handling mechanism and the local APIC. The SMI takes precedence over an NMI and a maskable interrupt. SMM is non-reentrant; that is, the SMI is disabled while the processor is in SMM.”
Many mainboard Chipsets (PCH), such as the Intel 500 series chipset family [2], expose the I/O addresses B2h and B3h, enabling the signaling of the SMI# pin on the processor. Writting a byte-value to the address B2h signals the SMI code that corresponds to the written value. The address B3h is used for passing information between the processor and the SMM and needs to be written before the SMI is signaled.
Chipsec [3] is the industry standard tool for auditing the security of x86 platform firmware. It is open source and maintained by Intel. Chipsec includes a module called smm_ptr, which searches for SMI handlers that result in the modification of an allocated memory buffer. It operates by filling the allocated memory with an initial value that is checked after every SMI call. It then iterates through all specified SMI codes, looking for changes in the buffer, the address of which is passed to the SMI via the processor’s general-purpose registers (GPRS).
Although highly useful as a reference approach to trigger SMIs by software, Chipsec’s smm_ptr module does not fulfill the objective of enumerating them. Only when the SMI has an observable change in the passed memory buffer does the module consider it vulnerable and flags its existance.
Since our goal is to enumerate SMIs, I considered measuring the time it takes for the SMI to execute as a simple measure of the complexity of its handler. The hypothesis is that an SMI code ignored by the BIOS would result in a shorter execution time compared to when the SMI is properly attended. With this objective in mind, I added the ‘scan’ mode to the smm_ptr module [4].
The scan mode introduces a new ioctl command to the Chipsec’s kernel module that triggers the SMI and returns the elapsed time to the caller. This mode maintains an average of the time it takes for an SMI to execute and flags whenever one exceeds a defined margin.
In the initial tests performed, an unexpected behaviour was observed in which, with a periodicity of one second, a ten times larger runtime appeared for the same SMI code. To confirm these outliers were only present when the SMI was signaled, I implemented an equivalent test measuring the time spent by an equivalently long time-consuming loop replacing the SMI call. The results of both tests are presented below.
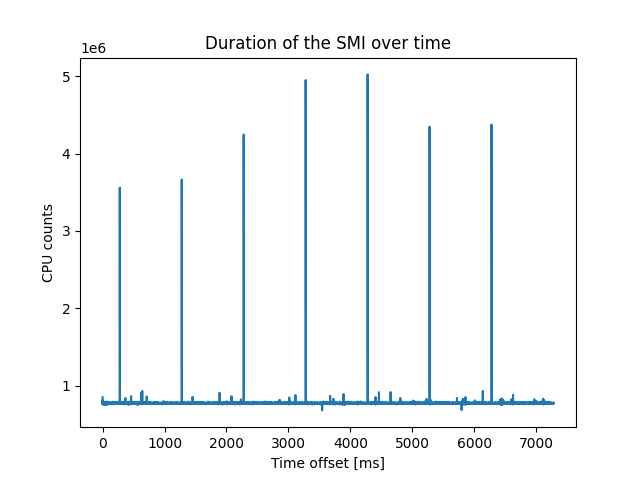
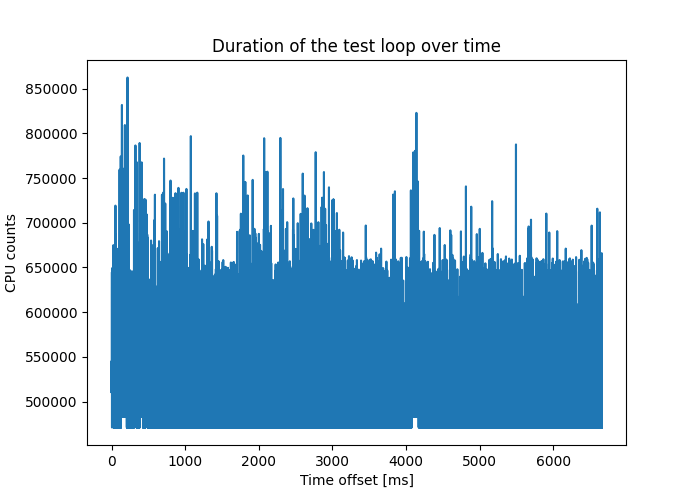
The details of each long-running SMI are detailed next, where ‘max’ and ‘min’ values are the maximum and minimum measured elapsed time in CPU counts, ‘total’ is the number of SMIs signaled, ‘address’ shows the register used for passing the address of the allocated buffer, and ‘data’ is the value written to the I/O address B3h.
SMI: 0, max: 5023124, min: 680534, count: 7, total: 12288,
long-running SMIs: [
{'time offset': 278.017 ms, 'counts': 3559564, 'rcx': 11, 'address': rbx, 'data': 0x09},
{'time offset': 1278.003 ms, 'counts': 3664844, 'rcx': 14, 'address': rbx, 'data': 0x2C},
{'time offset': 2277.865 ms, 'counts': 4244506, 'rcx': 1, 'address': rbx, 'data': 0x50},
{'time offset': 3277.685 ms, 'counts': 4950032, 'rcx': 4, 'address': rsi, 'data': 0x73},
{'time offset': 4277.681 ms, 'counts': 5023124, 'rcx': 8, 'address': rbx, 'data': 0x96},
{'time offset': 5277.898 ms, 'counts': 4347570, 'rcx': 11, 'address': rbx, 'data': 0xB9},
{'time offset': 6277.909 ms, 'counts': 4374736, 'rcx': 14, 'address': rsi, 'data': 0xDC}]
I don’t know the reason for these periodic lengthy SMIs. I can only speculate these might be NMI interrupts being blocked by SMM and serviced with priority right after exiting SMM and before the time is measured. In any case, I opted for performing a confirmation read once a long-running SMI is found, which effectively filters out these long measurements, resulting in the output shown below. It has an average elapsed time of 770239.23 counts and standard deviation of 7377.06 counts (0.219749 ms and 2.104e-06 seconds respectively on a 3.5 GHz CPU).
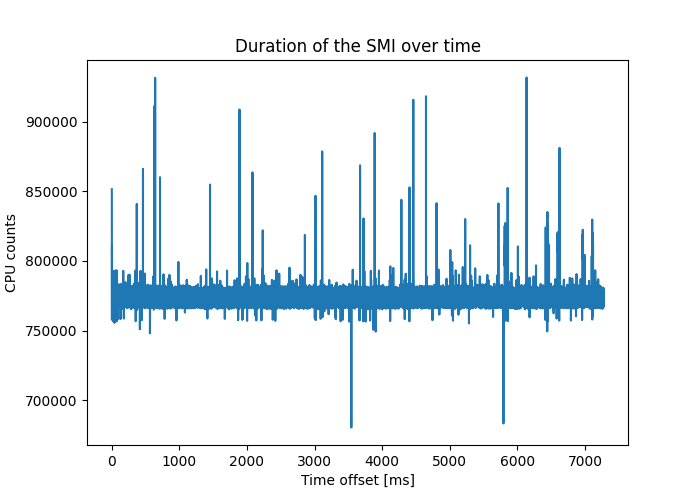
To discard any effects of the values passed to the SMI, I ran the test by repeatedly signaling the same SMI code and parameters. Below is the result using the confirmation read strategy, showing an average value of 769718.88 counts (0.219600 ms) and standard deviation of 6524.88 counts (1.861e-06 seconds).

The proposed scan mode is effective in identifying long-running SMIs present in the system. However, it is unable to find others that fall within the bounds of the defined threshold. For example, using an arbitrary threshold of 1/3 times larger than the average, the implementation was not successful noticing some of the SMIs flagged by the smm_ptr’s fuzz and fuzzmore modes. The main reasons are the large deviation observed and the challenge of dealing with a system for which no confirmed SMI codes are provided, making it difficult to calibrate the algorithm and establish a suitable threshold value.
The implementation has been merged into the upstream version of Chipsec and will be included in the next release [5].
[1] Intel® 64 and IA-32 Architectures Software Developer’s Manual Volume 3 (3A, 3B, 3C, 3D): System Programming Guide
[2] Intel® 500 Series Chipset Family On- Package Platform Controller Hub Datasheet, Volume 1 of 2. Rev. 007, September 2021.
[3] https://chipsec.github.io/
[4] https://github.com/nccgroup/chipsec/commit/eaad11ad587d951d3720c43cbce6d068731b7cdb
[5] https://github.com/chipsec/chipsec/pull/2141